- Homepage
- >
- Research
Research
Acid/Base dysregulation (NSF CAREER Grant)
Increased acidity in the body causes significant deleterious effects to bone health, including enhanced cancer invasion and associated bone pain, reduced bone density, increased fracture risk and functional limitations leading to compromised quality of life and mortality. Concerns about the effect of acidemia on bone health is only growing due to the prevalence of acidic diets, the rising number of chronic kidney disease and diabetes patients, and the aging population which are all correlated to decreased physiological pH. Despite the known negative consequences of acidemia on bone health, little is known about the mechanisms by which it affects bone quality and mechanical function. Therefore, my lab focuses on elucidating the complex relationship between the musculoskeletal system and acid-base balance in the body to inform improved preventative care and treatment modalities.
We are using a comprehensive 3 pronged approach to understand the biological and physiochemical processes responsible for bone degradation with acidosis. First, we have developed the only successful in vivo model of murine metabolic acidosis which recapitulates the bone loss and mechanical consequences seen clinically. This is paired with a combination of live and cell-free explant systems that allow us to isolate the physiochemical and cellular contributions of acidosis to bone loss. Lastly, we have developed one of the most comprehensive suites of biomimetic bone-like apatites to elucidate the chemical mechanisms responsible for mineral dissolution and buffering during acidosis.
Examination of these three exceptional systems using state-of-the art characterization techniques including Raman Spectroscopy, FTIR, uCT, histology, RNA analysis, high-energy synchrotron X-ray diffraction, and mechanical testing has allowed us to isolate the contributions of physiochemical and cellular bone responses to the disrupted pH. So far we have shown that contrary to the established paradigms, bone degradation during short term acidosis is primarily caused by physiochemical processes. This leads to changes in bone composition resulting in compromised mechanics. Our continued work on this topic is examining the ways in which (1) changes in bone mineral composition affect tissue mechanics (2) the acidic thresholds responsible for cellular activation and (3) the effect of varying treatments of bone recovery.
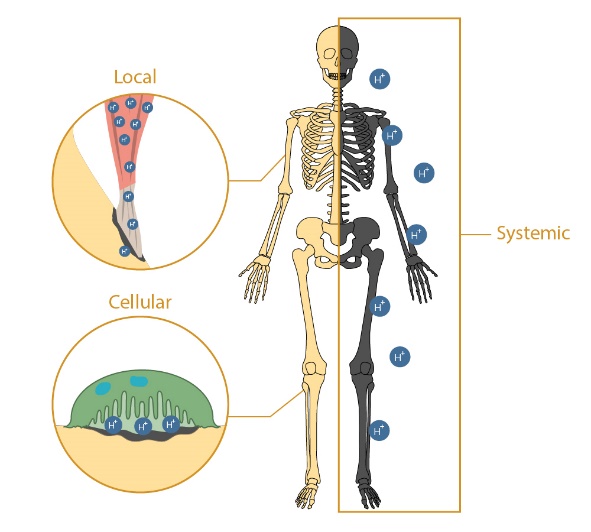
My lab focuses on elucidating the complex relationship between the musculoskeletal system and acid-base balance in the body to inform improved preventative care and treatment modalities.
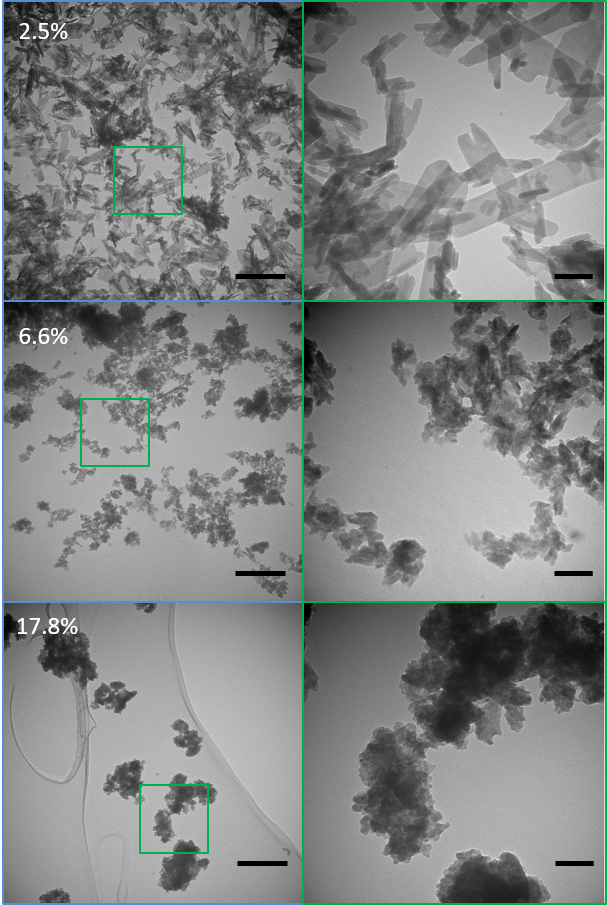
Our objective is to understand how changes in DEJ structure affect tooth fracture in DGI dentition in order to develop improved treatments and preventative care.
Dentinogenesis Imperfecta (NIH RO3)
Dentinogenesis imperfecta (DGI) is a genetic dental condition affecting ~1 in 7000 individuals worldwide that is characterized by dentin hypertrophy and loss of enamel. Without the enamel, teeth are at risk for rapid wear, fracture, and tooth loss. Despite these negative effects, the structural and mechanical cause of the enamel loss in DGI is unknown due to a lack of knowledge of how DGI affects the dentin-enamel junction (DEJ), the interfacial zone responsible for binding enamel to the underlying dentin. Thus, our objective is to understand how changes in DEJ structure affect tooth fracture in DGI dentition in order to develop improved treatments and preventative care.
We are using a hierarchical approach to examine the structure, composition and mechanics of the DEJ in healthy mice and those with DGI from our osteogenesis imperfecta colony. At the macro and micro-scale using high resolution Raman mapping, histology, μCT, and SEM we have shown so far that the healthy DEJ exhibits numerous graded structures at varying length scales that allow the DEJ interface to dissipate stresses. However, some of these gradients are not conserved in the DGI mouse pointing to possible mechanisms for enamel loss. At the sub-micro and nano-scale we are continuing the work by examining the DEJ structure and composition using SEM-FIB and TEM tomography.
Mechanically, we are using a combination of simultaneous macroscale compression testing, microscale digital image correlation analysis, and nanoscale high-energy synchrotron concurrent small and wide-angle X-ray diffraction measurements to simultaneously acquire information about macroscale mechanical properties, microscale interfacial tissue deformation, and nanoscale collagen and mineral strain, respectively. This unique hierarchical mechanical testing system will allow us to correlate multi-scale variations in structure and composition to equivalent scale mechanical changes. A combination of numerical and analytical computational modeling techniques will be used to coalesce the compositional, structural, and mechanical data into a single comprehensive and cohesive mechanical model of the DEJ. This modifiable model will serve as the first tool for predicting DEJ responses to loading and pathological changes.
Calcific Tendinopathy (DoD DEPSCoR Grant)
Mineral can appear in the body both in healthy hard tissues but also as pathological deposits in soft tissues. Calcific tendinopathy is an example of pathological mineralization where bioapatite is deposited in tendinous tissue leading to increased pain, disability, and tendon rupture rates. Despite these complications, the mechanism by which mineral grows within the tendon remains unclear. The deposition of bioapatite is generally described as being thermodynamically unfavorable according to classical thermodynamics. However, traditional thermodynamics fails to account for the effects of applied stresses that have been shown to create unexpected conditions at equilibrium. With the increased incidence of tendon mineralization in athletes pointing to the importance of loading, my lab has focused on developing an experimentally validated field-driven thermodynamics framework to address the interplay of stress fields with electrochemical reactions, and their ensuing effects on the compositional and microstructural evolution of mineralization during tissue deformation.
We are using a combined experimental and in silico approach to elucidate the role of applied stress on the growth and composition of bioapatite. We are developing a unique thermodynamics model of bioapatite in equilibrium with body fluids under stress considering both mechanical and electrochemical factors evolving from the work of Larché and Cahn. By inputting our extensive knowledge of apatite properties into the model we will predict the effect of stress on mineral composition, dissolution, and growth. So far our preliminary work has shown that applied load promotes “unfavorable” carbonate inclusion and apatite crystal growth suggesting that load may be a major contributor in mineralization. Experiments using synchrotron XRD to probe lattice strain and growth of bioapatite mineral in an aligned tendon-like collagen scaffolds under load will be used to validate the theoretical model. This will not only provide a unprecedented model for predicting the effect of loading on bioapatite growth and composition in a biological environment but can be used to make extraordinary predictions on the mechano-chemical environments necessary to promote or reverse mineral growth in tendon-like tissues facilitating the optimization of mechanics and chemistry-based treatments for calcific tendinopathy.
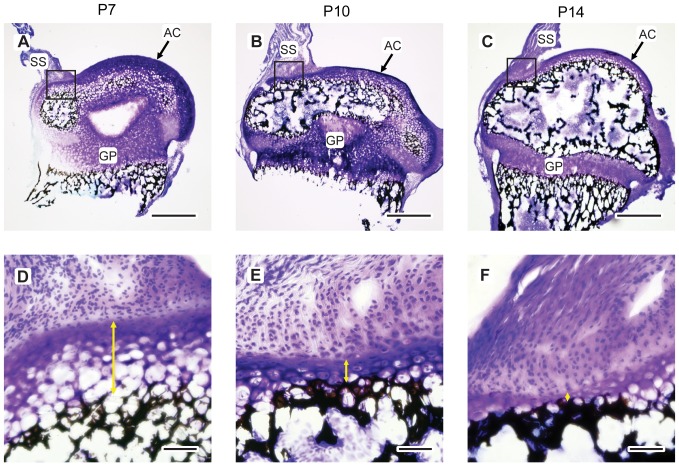
My lab has focused on developing an experimentally validated field-driven thermodynamics framework to address the interplay of stress fields with electrochemical reactions, and their ensuing effects on the compositional and microstructural evolution of mineralization during tissue deformation.